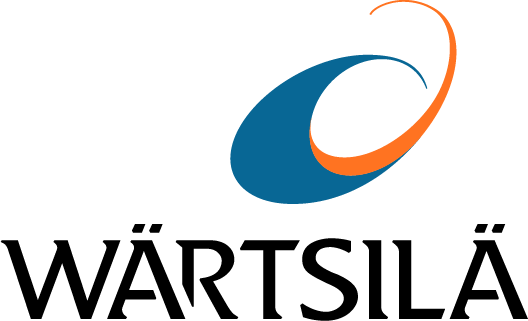
Throughout the technology comparison article series, we are delving into various aspects of reciprocating internal combustion engines and aeroderivative gas turbines. Although both technologies are considered flexible, combustion engines can start and stop more quickly, adjust power more rapidly and operate more efficiently at various loads and ambient conditions compared to aeroderivative gas turbines. To put it another way, combustion engines are more efficient, flexible and future-proof.
Chapters 3 and 4 of this series focus on key attributes that enable the efficient integration of larger quantities of renewables while increasing the flexibility and reliability of the power system. The output of variable renewable energy sources is rapidly increasing, and this variability is seen as a challenge for power systems. However, flexibility is key. The faster and more flexible the technology, the better it will support the buildout of renewable capacity and address the challenges that intermittency poses. Nevertheless, this cannot be done at any cost, which is why we must also pay attention to plant net efficiency.
Table 1. Six elements of dispatchability.
Technology | Start-up time to full load (minutes) | Ramp rate (in spinning mode, %/min) | Min. uptime (minutes) | Min. downtime (minutes) | Min. stable load (emission compliant) | Plant net efficiency, LHV |
---|---|---|---|---|---|---|
Combustion engine | 2–5 | >100 | 0 | 0 | 10% | 45–48% |
Aeroderivative gas turbine | 5–8 | 50 | 10–30 | 30–60 | 40% | 37–40% |
Table 1 shows how combustion engines and aeroderivative gas turbines differ. Preheated engines can reach full output in two minutes and their ramp rate from spinning mode exceeds 100 per cent per minute. There are no limitations on minimum uptime or downtime, and the minimum emissions-compliant stable load of a single unit is only 10 per cent of the full output, which is why engines are capable of repeated ramp ups and ramp downs without incurring additional maintenance.
Chapter 4 discusses plant net efficiency for multi-unit power plants across a plant load range. The capability to provide a range of incremental part loads without sacrificing efficiency is one key aspect of plant net efficiency, as market experience demonstrates that flexible gas power plants operate at varying loads for economic reasons.
Part-load efficiency matters because it helps to minimise fuel costs and emissions and maximise operational flexibility since the operating regimes of thermal power plants are changing from pure baseload to balancing renewables. A power plant can consist of multiple independent units, which is why considerations such as part-load efficiency and efficiency at varying temperatures at the unit level are important topics, as discussed in chapters 5 and 7.
Figure 1 presents the net efficiency of a multi-unit engine power plant with a maximum plant output of approximately 100 MW at varying load levels. The plant demonstrates superior performance across the full plant load range as combustion engines have a low minimum stable load and high efficiency at part load. In addition, they can maintain high performance in different ambient conditions since high ambient temperature has no impact on engine output and only a minor impact on efficiency. The plant consists of 10 independent units, enabling the efficient use of a subset of the plant capacity and offering a wide load range of 1–100 per cent.
Figure 1: Plant net efficiency at varying load levels.
Performance figures typically represent full load operation for new equipment at 15°C (59°F) ISO conditions, resulting in overly optimistic net efficiencies for local conditions and the actual operating profile. Therefore, there are many operational factors that must be considered when assessing the actual net efficiency of a power plant.
Firstly, power plants often operate under varying ambient conditions, and seasonal temperature changes can be significant, varying by tens of degrees, making a 15°C (59°F) temperature reference point irrelevant in terms of the actual net efficiency.
Secondly, in balancing applications, power plants experience lower operation times and average loads compared to baseload, where high annual operating hours and full stable output are common.
Thirdly, degradation occurs over the lifetime of equipment, leading to reduced output and efficiency compared to newly installed equipment. Furthermore, an increasing number of equipment starts, stops and restarts consumes more fuel compared to operating steadily at a stable load, further reducing efficiency and resulting in additional maintenance. All these factors collectively impact real-world net efficiency.
Figure 2 A: Actual net efficiency considering several operational factors for combustion engine.
Figure 2 B: Actual net efficiency considering several operational factors for aero.
Figure 2 shows the impact of these separate operational factors and the difference between actual net efficiency after adjustments and reference full load performance at ISO conditions. As the figure depicts, combustion engines already have a head start at the reference point, but they can also outperform aeroderivative gas turbines, offering superior real-world net efficiency. The substantial disparity in efficiency between combustion engines and aeroderivative gas turbines translates into a significant fuel saving potential of nearly 25 per cent.